Physical Changes
During a physical change, no new substances are formed.
Although the properties may change, the particles present never change.
Generally physical changes
- require moderate amount of energy,
- are easy to reverse and
- do not result in a change in chemical identity.
A typical example of a physical change is any change
in state, which simply requires the addition or removal of energy.
These processes are good examples of physical changes since they involve
very little energy, do not produce a new material and can easily be reversed
by either adding or removing energy. The term freezing and melting specifically apply to water; for other substances, the terms solidification and liquefying should be applied respectfully. If the change from a liquid to a gas occurs with the addition of heat to raise the temperature of the liquid above its boiling point, the change is actually called vapourization. For example, on a warm sunny day, a puddle of water will experience evapourate which will make the puddle slowly disappear. However, the puddle does not vapourize since we do not see it boil. In the lab, when we heat up water with a Bunsen burner to convert the water into steam (actually a mixture of liquid and gas) and then into gaseous water, the process is vapourization. It is a qualitative distinction in many regards, but an important one to note. Sublimation from a gas to a solid can also be called deposition or crystallization.
Physical changes occur when the attractions BETWEEN particles is affected. These attractions are weak and that is why little energy (compared to a chemical change) is required. The particles in a solid are only undergoing vibration and this allows the maximum number of attractions to exist at any one movement. As a result a solid is a very rigid structure with a constant volume and shape. When a solid melts, it becomes a liquid as the attractions between the particles are weakened due to the addition of energy. As a result, the particles
spread out, but they are still the same particles. Ice is
made up of particles of water. When it melts the water which is formed
is made up the same water particles. However, the additional energy made the particles in the solid move more which broke some of the attractions between particles and now the particles can vibrate and rotate around in space. This is why a liquid does not have a constant shape (takes the shape of the container). The particles in the liquid still have a considerable number of attractions between them so they can't go far from each other and thus a liquid does have a constant volume. As more heat is applied to the liquid, the particles eventually possess so much energy that they break most of the attractions between the particles allowing the particles to move from one location to another in addition to rotational and vibrational motion. This is now the gas state.
When a solid melts, it becomes a liquid and the particles
are more spread out, but they are still the same particles. Ice is
made up of particles of water. When it melts the water which is formed
is made up the same water particles and when it boils the steam is also
made up of the same water particles. Particles stay the same unless
there is a chemical change whether the matter is solid, liquid or gas.
Only their arrangement, energy and movement changes. When substances change state there is no change in mass
so if 100 g of ice is melted 100 g of water are formed this will boil to
form 100 g of steam (this is called "conservation
of mass"). If this steam could be collected, cooled and
condensed it would form 100 g of water which could be frozen to give 100 g
of ice. Students sometimes think that solids get lighter when they
melt as liquids are lighter than solids; however, this is not true.
Dissolving is also a physical process because it
is reversible represented by considering particles mixing closely together
and reversed by separating the particles. Dissolving is nothing more
than separating the particles within the solid to make them spread apart
to form a liquid. It is reversible because if the particles are forced
back together (as can happen when you vapourize the liquid they were dissolved
into) to recreate the solid. The shape of the solid may not be the
same (e.g. it would be hard to reform a sugar cube in this manner), but
the particles would be the same (i.e. it would still taste like sugar).
Chemical Changes
During a chemical change, at least one new
substance is formed with new properties. Generally chemical changes
- involve larger change of energy,
- are generally less easy to reverse and
- result in a change in chemical identity.
Chemical changes involve a change in chemical composition,
but these changes takes place at the particle level.
These changes are not easily reversible.
If you add or remove heat, you will not get the same material back.
For example, if you fry an egg and then cool it down, it will not return to its
original runny-like material. These chemical changes permanently altered the
particles (in this case the particles changed shape and formed new groups). Chemical changes alter the attractions within the particles. There are three ways a chemical change can happen:
- particles can combine to form a new particle
- a particle may split into component particles (can't split atoms though)
- a combination of the above two processes can happen in one reaction
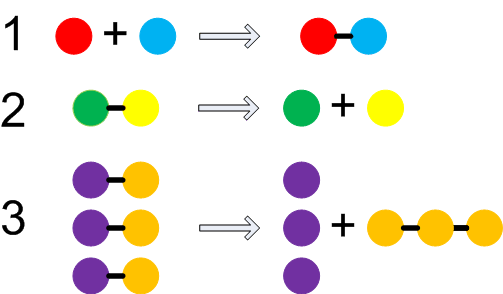
Often some energy must be supplied initially to initiate the process. Typical chemical
changes are most likely to be encountered while cooking and during combustion
(burning). Changes due to cooking are often complex as the substances
contained within foods are complex. Common examples include eggs
and cake mixtures going hard when they are cooked or potatoes and other
vegetables going soft. The key question is, how do you know that a chemical change has taken place? Generally, we look for signs of a change in the composition of matter. This means a new substance was formed or a previous substance disappeared. However, these are not clear indicators of a chemical change since they can easily look like a physical change. For example, how do you know a solid reacted or simply dissolved. Or how do you know a precipitate formed or the solvent simply evapourated leaving a solid behind. We must rely on more conclusive evidence of chemical change. These signs are:
- a gas is produced which was not the result of addition of heat
- the temperature changes, but not due to external changes
- a solid (precipitate) is formed (not just from evapouration)
- a colour change occurs (not just mixing)
But it is not always easy to see exactly what has
happened during a chemical change. Sometimes the reactants (starting
materials) and products (end materials) may be difficult to see especially
if they are gases. For example when fuel burns it reacts with oxygen
gas (from the air) to produce water vapour/ liquid and carbon dioxide gas.
So within this reaction there are two reactants and two product and two
of these are colourless gases (oxygen and carbon dioxide) and the
water formed as a result of the burning may be formed as steam and be difficult
to see. Consequently all that would be observed apart from the flame
is the disappearance of the fuel. As a result, we usually prefer to see more than one sign that a chemical change has taken place. However, we cannot be absolutely sure that a chemical change has taken place until we analyze the chemical composition of the products of that change. The products should have different physical properties (such as boiling point) than the reactants.
What is a Chemical Reaction?
A chemical reaction represents the exchange of atoms/molecules resulting in a new combination of atoms/molecules. It can result in the joining of particles to make a new particle or the splitting of a particle to make new particles or rearrangements of some particles to create new arrangements or some combination of these events. Energy is always involved and is the driving force.
The key indicator of a chemical reaction is that new material or materials are made. This does not mean that new elements have been made; that would take considerable amounts of energy and nuclear rearrangement. Converting one element into another is not magical, but it is extremely difficult and takes considerable time and energy. This was the goal of the alchemist (the first form of chemist) who sought to convert inexpensive elements such as lead into expensive elements such as gold.
A chemical equation is a way to describe what goes on in a chemical reaction. Chemical equations are written with the symbols of reactants made up of atomic symbols. There is an arrow pointing in the direction of the reaction. The materials to the left of the arrow are the reactants, or materials that are going to react. The materials to the right of the arrow are the products, or materials that have been produced by the reaction. There are such things as reversible reactions, reactions in which the products reassemble to become the original products. Reversible reactions are symbolized in chemical equations by a double-headed arrow, but the standard remains to call the materials on the left the reactants and the materials on the right the products.
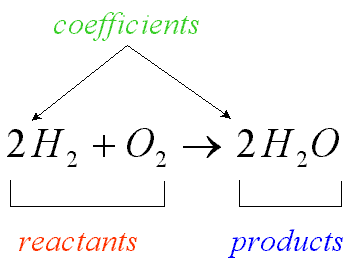
Special Chemical Reactions
A study of chemical reactions always starts with some very general types of reactions to serve as a basis for understanding more complex chemical interactions. It may appear that so much attention is typically devoted to these general introductory chemical reactions that the more complicated, specific chemical reactions are fewer in number or so complicated that they are unique and thus, can only be studied individually. This is far from the truth in both cases. The number of specific chemical reactions is indeed massive with more being defined each day; however, they still can be classified and clumped into groups based on key features.
A very common, specific reaction is an oxidation-reduction reaction or redox reaction. Redox reactions involve a transfer of electrons and can be identified when one chemical has lost electrons to become more positively charged while another chemical has gained electrons to become more negatively charged during a chemical reaction. Since this can occur during any reaction, this is really just a different way to classify some reactions. Oxidation and reduction are two opposite but complementary processes; one cannot occur without the other since electrons cannot simply disappear or appear. Oxidation occurs when atoms lose electrons while reduction occurs when atoms gain electrons. An easy acronym to remember this is LEOGER which stands for Losing Electrons is Oxidation; Gaining Electrons is Reduction or OILRIG which stands for Oxidation is Losing Electrons; Reduction is Gaining Electrons.
Oxidation originates from the fact that in nature oxygen is the most abundant and effective element stripping electrons from other chemicals and so many oxidation reactions (but not all) rely on oxygen. Reduction has more of historical context in that many mined ores such as iron become lighter in mass as they gain electrons (e.g. FeO is lighter in mass than Fe2O3) but this is not always true for other chemicals. Since redox also occurs during other reactions, the driving force and destabilization event is largely determined by the other reaction type (combustion, synthesis, decomposition, displacement, etc.). However, if only a redox occurs, the driving force is simply bringing reactants into close proximity to allow attraction of electrons. The destabilization event is the difference in potential to gain or lose electrons. Although little energy is needed, it would aid in this process. The process in not typically reversible because the products represent a lower energy state (more stable) or are locked in (solids, liquids and gases). The best way to keep track of electron exchange is by finding the net ionic equation. Consider the reaction between copper(II) sulfate and solid zinc:
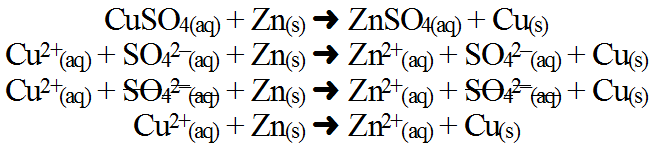
In this reaction, destabilization succeeds because Zn(s) has a stronger ability to lose electrons than Cu (see the activity series), thus, Zn(s) loses 2 electrons (oxidation) to change its oxidation number from 0 to 2+ while Cu2+(aq) gains those electrons (reduction) to change its oxidation number from 2+ to 0. Since Zn(s) forces Cu2+(aq) to accept electrons, Zn(s) is called the reducing agent and since Cu2+(aq) allows Zn(s) to lose electrons, Cu2+(aq) is called the oxidizing agent. Identifying these chemicals must be extremely specific as there is a big difference between Zn(s) and Zn2+(aq). Typically oxidation and reduction reactions are written by themselves in what are called half-reactions showing electrons gained and lost. Usually the net ionic equation is used. In the example above, the half reactions would be (note that it helps to keep the arrows lined up):

Notice the oxidation had electrons being lost as a product while reduction has electrons added as a reactant. In the end, the half-reactions work simulataneously to ensure that the number of electrons lost by one reaction exactly equals the number of electrons gained by the other reaction such that electron exchange is cancelled out of the final summative equaction. Now consider another example which you might think would cancel since everything is aqueous, but it actually works:

So we see that this reaction occurs because the oxidation numbers have changed. It would look like a subtle change in colour in this case. Look at the half-reactions:

Now consider a hard, but very typical specific redox reaction:

There are no spectator ions so there is no ionic equation so you must work with changes in charges more directly. Note the Fe(s) and O2(g) have an oxidation number of 0 (no charged) and while Fe2O3(s) has an overall oxidation number of 0, the elements within the compound do have oxidation numbers which you know from nomenclature and uncriss-crossing. This is where the half-reactions work best.

Another common specific chemical reaction is a reaction which results in the production of a new acid or base. This is much more than simply adding an already existing acid or base to water; this is the actual creation of a new acid or base compound. The first surprise is that production of acids and bases is actually trickier than people realize. They are soluble and highly reactive so in order to have an acid or a base; that is, aqueous H+ or OH-, readily available and capable of interacting freely with other chemicals means they can’t just stored very long as they would interact with their surroundings and would no longer be available. New acids/bases can be made from direct reactions to a limited degree and this is fine for laboratory work and does rarely occur in nature. Consider to more common examples of these types of direct acid/base creation reactions.
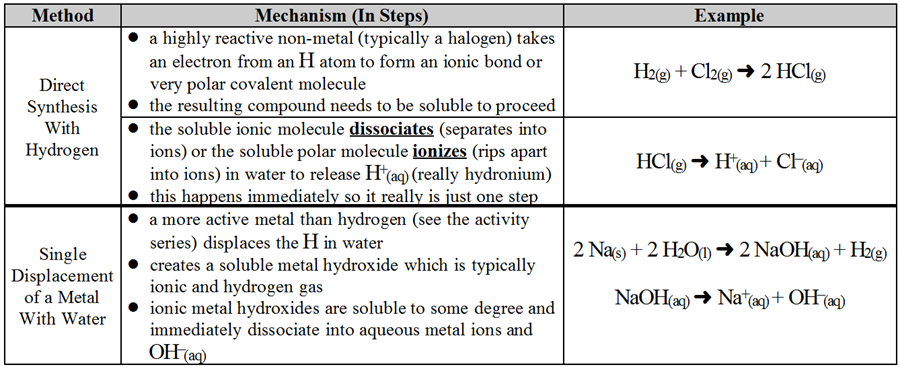
However, this is not the case for natural systems such as in our bodies or the environment. In nature, the chemicals required for direct synthesis are not common, the process is quite energy-demanding and the acids/bases formed are far too corrosive for safe and long-term storage within organisms or in the environment. These reactants needed must be easily produced as needed which means a plentiful source of reactant that does not produce these ions too easily (so it persists until needed) is required. 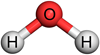
A more stable and common method relies on using water as an actual reactant. Water is plentiful and naturally breaks down into both H+(aq) and OH-(aq). However, this self-ionization or autolysis of water molecules is too slow and cannot change acidity of basicity of the resulting solution since it releases equal numbers of H+(aq) and OH-(aq). Thus, there needs to be a way to alter the final ratio of H+(aq) and OH-(aq) forcing the final solution to become acidic (more H+(aq) and OH-(aq)) or basic (less H+(aq) and OH-(aq). In nature, oxygen appears to be the key as it is abundant and reactive enough to speed up the rate of water breakdown while also altering the ratio of H+(aq) and OH-(aq) leaving the final solution either acidic or basic instead of neutral.
So the task is to find oxygen-containing compounds strong enough to break force autolysis of H2O into free aquesous H+ and OH- but another challenge is that this other chemical can’t be too reactive on its own or it won’t persist until needed and still one more challenge is that in the end, this other chemical must ensure that only one of either H+ and OH- continues to exist or the result would still be neutral water as there would be equal amounts of acid and base present. The compounds that serve these goals are the oxides. They are incredibly stable but contain a reactive oxide ion which is capable of breaking water apart. So we are looking as specific reactions involving oxide formation, interaction with water and finally, acid or base creation.
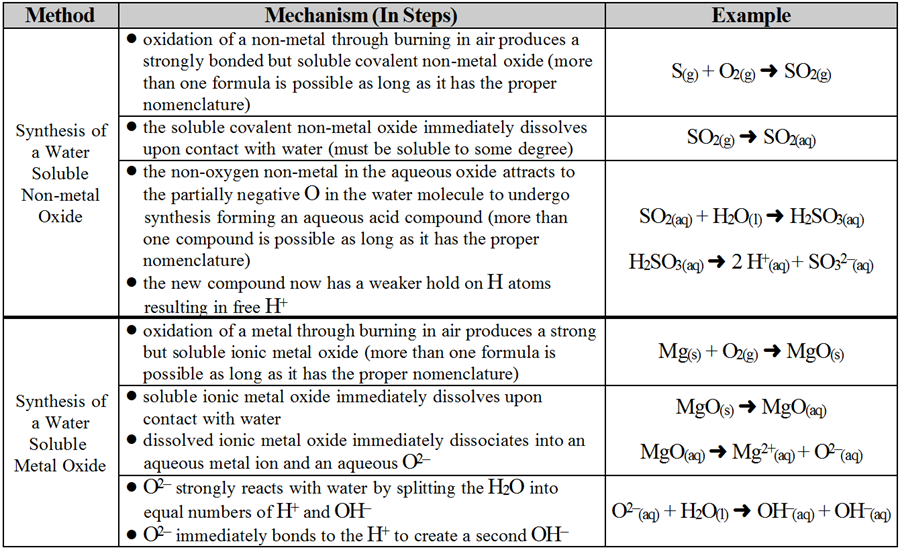
The more soluble the non-metal oxide and/or the more non-metallic the non-metal, the stronger the acid produced. Likewise, the more soluble the metal oxide and/or the more metallic the metal, the stronger the base produced.
References
http://www.chemtutor.com/react.htm#what
http://www.mikeblaber.org/oldwine/chm1045/notes/Stoich/Equation/coeff.gif
http://boomeria.org/chemtextbook/fig16-9.jpg
http://www.elmhurst.edu/~chm/vchembook/images2/171saltdissolve2.gif
http://chemsite.lsrhs.net/Intro/images/Overheads_large/classification_of_matter.jpg
http://img.sparknotes.com/content/testprep/bookimgs/sat2/chemistry/0003/sat117002_0503.gif