Numbers
To be able to use quantitative physical properties, it is important to be fluent with the metric system. The following is a table of the most common prefixes:
The following figure is useful for metric conversions:
Typicall scientific numbers are so large and require high levels of precision. As a result, scientific notation is often used. The rules are
Rules for Scientific Notation
- non-zero digit is placed before the decimal
- about 2-5 digits are placed after the decimal
- "X 10" to the appropriate exponent is placed after the number--if you have to move the decimal to left, exponent is more
positive and if you move decimal to right, exponent is more negative
e.g. express the following numbers in scientific notation:
- 1994 = 1.994 X 103
- 0.051 = 5.1 X 10-2
- 6.75 = 6.75 not 6.75 X 100as 100 is always omitted
Note that the EXP button on calculator = "X 10" thus hit EXP before typing the exponent (if you don't have a calculator, convert all numbers to same
exponent and subtract exponents if dividing, add exponents if multiplying or keep same exponent if adding/subtracting).
Classification
The need to classify is inherent in humans.
It makes our lives easier by allowing us to know something about a wide
variety of things and allows us to make predictions about things we do
not know much about. Classification is usually part of the early
stages of understanding science. It allows us to sort our data into
unifying categories so that we can wrestle with "what it means" in a more
efficient manner. A chemist will usually classify all "stuff" that
has "substance", i.e. matter. The classification scheme currently
used, although seemingly straightforward, is the result of centuries of
investigation and thought! Matter can be described empirically (directly observed) or inferentially (implied based on properties). Empirical is typically where we start since these descriptions or definitions only rely on our senses to understand the world.
One way to describe matter empirically is by using a structural definition in that the matter is observed in an unchanging condition and described. Consider the states of matter.
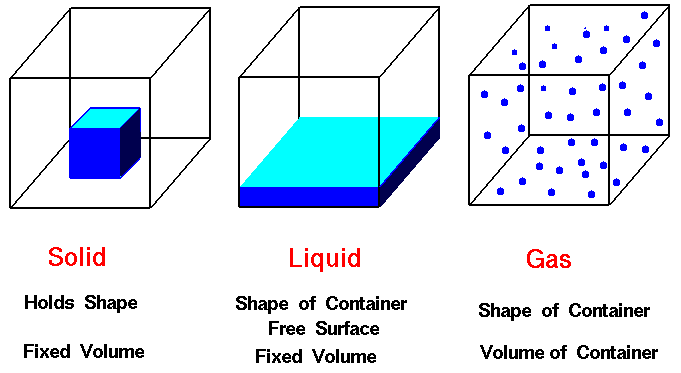
All substances have properties that we can use to
identify them. For example we can identify a person by their face,
their voice, height, finger prints, DNA etc.. The more of these properties
that we can identify, the better we know the person. I n a similar way
matter has properties - and there are many of them. There are two
basic types of properties that we can associate with matter. These properties
are called physical properties (do not change the chemical nature
of matter) and chemical properties (do change the chemical nature
of matter). Physical properties can be classified as quantitative (measurable) or qualitative (not measurable), but more importantly they
can be identified as either intensive (do not depend on the amount of the
matter present) or extensive (change with the amount of matter present). Examples of intensive properties include color, odour,
luster, malleability, ductility, conductivity, hardness, melting/freezing
point, boiling point and density. Examples of extensive properties
include mass, weight, volume or length. Examples of chemical properties
are: heat of combustion, reactivity with water, pH, and electromotive force.
Chemical properties tend to also be operational properties in that they can only be observed as a result of change. For example, you cannot tell just by looking at iron or aluminum that they rust, but you can expose them to oxygen and over time see that they do indeed rust. Therefore, you know can state that iron is a metal capable of rusting (not all metals rust).
The more properties we can identify for a substance, the
better we know the nature of that substance. These properties can
then help us model the substance and thus understand how this substance
will behave under various conditions. The most useful properties
are those which are quantitative and intensive because they do not require
you to change the substance and they do not depend upon the amount of the
sample you possess.
However, classification is really more inferential since we are trying to make sense of our observations.
On the simplest level, matter is that which
is not energy. Einstein proposed that the two were just extremes
of each other and convertable through the equation of E=mc2.
However, this relationship is anything but simple. Energy, it seems,
isn't made up of any one thing; instead, it's an abstract concept that
is popularly defined as "the ability to do work". But if you really
want to force a breakdown of energy you could say, for example, that radiation
comes in discrete clumps called photons. These can be thought of as making
up radiative energy. Similarly, interconversions between the bonds
and structures of atoms and molecules can be thought of as giving rise
to chemical energy. Electrical energy depends on the movement of
electrons. Heat energy can be transmitted via radiation conduction,
or convection. Conduction depends on molecules/atoms being close
to each other and, similarly, convection relies on a interaction of atoms
or molecules. But the general classifications like Potential Energy
(energy of position) and Kinetic Energy (energy of motion) can't really
be broken down.
The conversion between matter and energy isn't anywhere
near as simple as you would think because they're related on a fundamental
level such that one is the other! As it turns out, matter is every
bit as abstract as energy and the ethereal energy that you hear about is
as tangible as the matter you interact with! At their core, the "particles"
that make up everyday matter are really not anything like the solid spheres
we all tend to imagine. And energy itself is a lot more ubiquitous and
intrinsic part of our lives than we usually consider. But what does
the equivalence of matter and energy expressed by his famous equation E=mc2,
really
mean? It means that, at the fundamental level, there really is no
difference between energy and matter. One is equivalent to the other.
So what does this mean to you? It means that
using this definition of matter is good for making gross generalizations,
but that is as far as you can go. This philosophical definition remains
cerebral and useless in any real practical sense. Another classification
system is needed.
Our most useful classification systems tend to be specific and conceptual (based on what we think are underlysing reasons). Conceptual definitions are preferred since distinguish types of matter and allow predictions based on characteristics.
For example, reconsider the typical classification of states of matter, but now do so in terms of how the
particles are arranged. A solid has slow moving particles due to strong interparticle attractions forcing the substance to maintain
its shape and volume. A liquid, with its slightly faster moving particles
has slightly weaker interparticle attractions allowing shape to vary and a gas with its extremely fast moving particles
preventing any definite shape or volume since there are virtually no interparticle attractions.

From this we can define other conditions of matter
based on how the particles are arranged.
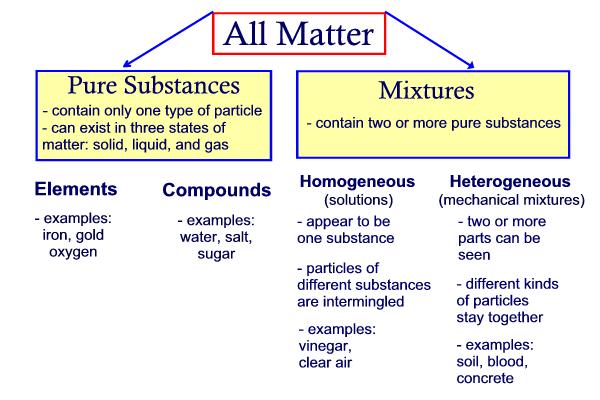
Note that the difference between a solution and
mixture depends upon the level of magnification and distribution of
the particles. Macroscopically solutions are very uniform while
mixtures are not. Microscopically solutions may not be as uniform
as predicted, but this will not be detectable. Mixtures demonstrate
varying properties due to their macroscopic non-uniform distribution.
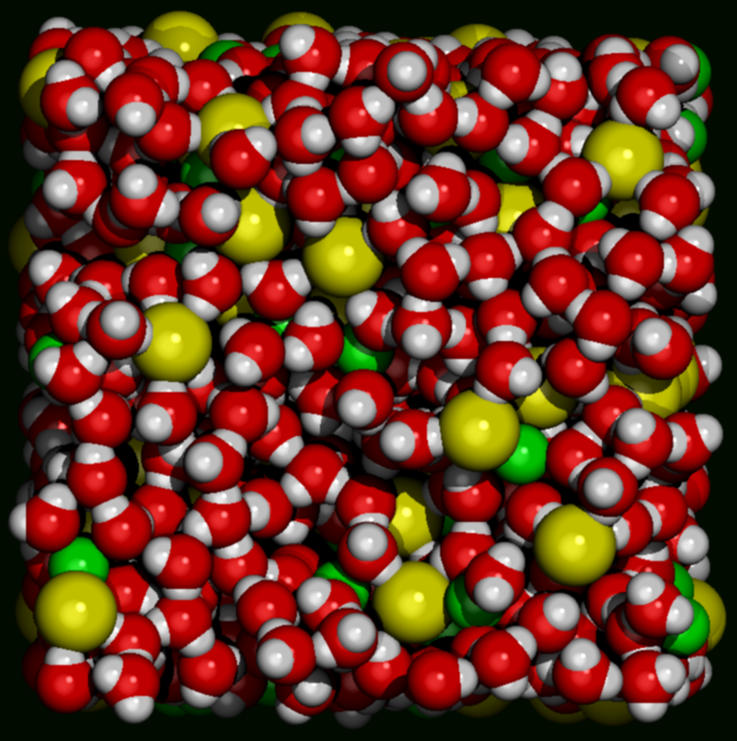 |
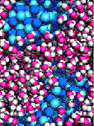 |
Solution |
Mixture |
The more specific the classification system
the more useful it becomes, but the more restrictive it becomes as
well. Thus the operational classification with properties such
as density and solubility and colour tend to be most used in the laboratory,
but require considerable work to explain on an atomic level.
References
http://dept.physics.upenn.edu/courses/gladney/mathphys/subsubsection1_1_3_1.html
http://www.madsci.org/posts/archives/nov98/912178539.Ph.r.html
http://www.sickamongthepure.com/files/Graphics/Library/E_MC2.jpg
http://www.chem.tamu.edu/class/majors/tutorialnotefiles/intext.htm
http://chemsite.lsrhs.net/a_Intro/images/Overheads_large/classification_of_matter.jpg
http://www.edquest.ca/Notes/clofmat.jpg
http://itl.chem.ufl.edu/2045/matter/FG01_005.GIF
http://www.ilpi.com/msds/ref/gifs/statesofmatter.gif