Defining Acids and
Bases
There are several methods of defining acids and bases.
While these definitions don't contradict each other, they do vary in how
inclusive they are. Initial definitions were based solely on how
acids and bases behaved or operated . These definitions were mostly
qualitative in nature, but were not really formal definitions. Several
scientists contributed to these definitions:
In 1661, Robert Boyle described acids and bases by
very simple characteristics:
Acids:
-
Sour taste
-
Corrosive
-
Change litmus (dye extracted from lichens) from blue to red
-
Become less acidic when combined with alkalies.
Alkalies (Bases):
-
Feel slippery
-
Change litmus from red to blue
-
Become less alkaline when combined with acids.
Around the same time, Antoine Lavoisier stated that all acids contained
oxygen after studying several acids such as sulfuric and nitric acid.
It was not until 1881, that Lavoisier's work was
formally questioned. Humphry Davy noted that hydrochloric acid did
not contain oxygen yet is an acid. Soon thereafter, several more
acids without oxygen were found such as hydrobromic and hydroiodic acid.
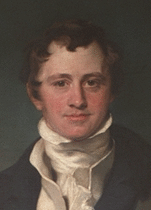
In 1838, Justus von Liebig suggested that acids
contain one or more hydrogen atoms which can be replaced by metal atoms
to produce salts. For example HSCN is an acid because the H atom
can be replaced by a metal to form a salt, such as NaSCN.
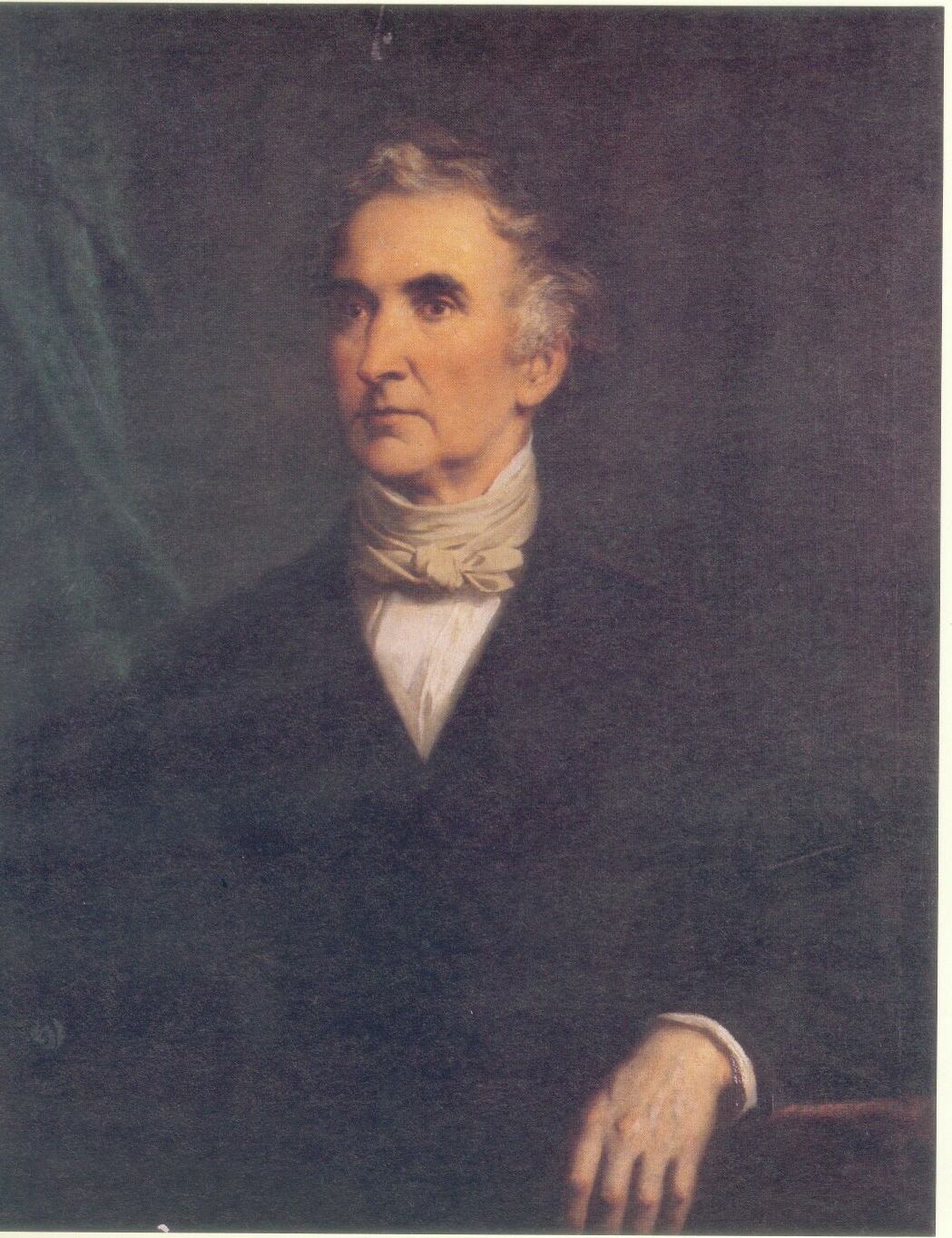
Although Justus von Liebig was the first to suggest that acids and bases
can be more formally defined, actual conceptual definitions were not formally
presented until 1884 when Svante Arrhenius proposed that salts dissociate
when they dissolve in water to give charged particles which he called ions
and in 1887, he extended this his idea by defining acids and bases as the
following:
-
Arrhenius acid - Any substance that ionizes when it dissolves in water
to give the H+ ion.
-
e.g.

-
Arrhenius base - Any substance that ionizes when it dissolves in water
to give the OH- ion.
-
e.g.

Arrhenius's theory helped to explain why acids have
similar characteristics, since they all give H+ ions when they
dissolve in water. It also explained why acids are neutralized by
bases and why bases are neutralized by acids; the H+ ions
from acids combine with the OH- ions from bases to form water:
Though the Arrhenius theory helped to explain more
about acids and bases, there were still several drawbacks to this theory.
-
The theory can only classify substances when they are dissolved in water
since the definitions are based upon the dissociation of compounds in water.
-
It does not explain why some compounds containing hydrogen such as HCl
dissolve in water to give acidic solutions and why others such as CH4
do not.
-
The theory can only classify substances as bases if they contain the OH-
ion and cannot explain why some compounds that don't contain the OH-
such as Na2CO3 have base-like characteristics.
To extend the Arrhenius theory a little further, consider
the formation of water from the combination of an H+ ion and
an OH- ion as a dynamic equilibrium:
Based on the fact that the above reaction is
reversible, we can conclude the following:
-
and acid is any substance that increases the concentration of the
H+ ion when it dissolves in water.
-
a base is any substance that increase the concentration of the OH-
ion when it dissolves in water.
Now, substances not containing H+ or OH-
ions can be classified as acids or bases if they alter the [H+]
or [OH-] when they dissolve in water. For example
CO2 cannot dissociate to give H+ but it does increases
[H+] when it is dissolves in water.
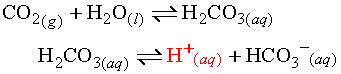
Likewise CaO cannot dissociate to give OH-
but it does increase [OH-] when it dissolves in water.

In 1923 Johannes Brønsted and Thomas
Lowry extended the understanding of acids and bases as they separately
proposed a new set of definitions for acids and bases which are known as
either Brønsted acids and bases or Brønsted-Lowry acids and
bases.
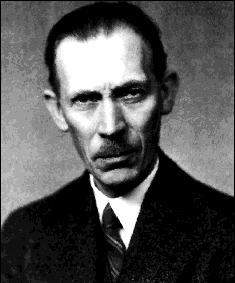
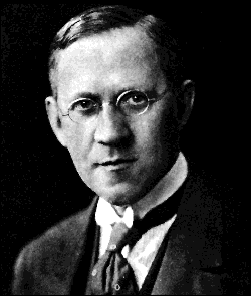
-
Brønsted Acid - Any substance that can donate a proton, H+
ion to a base. That is, it is a hydrogen-ion donor or proton donor.
-
Brønsted Base - Any substance that can accept a proton, H+
ion from an acid. That is, it is a hydrogen-ion acceptor or proton
acceptor .
In the above reaction, the H from HCl is donated
to H2O which accepts the H to form H3O+,
leaving a Cl- ion. The Brønsted-Lowry model of
acids and bases brings rise to the concept of conjugate acid-base pairs.
The part of the acid remaining when an acid donates a H+ ion
is called the conjugate base. The acid formed when a base accepts
a H+ ion is called the conjugate acid.
For the generic acid HA:
For the generic base A-:
More examples of conjugate acid-base pairs:
In the following reactions, it is shown how H2PO4-
and H2O can act as both acids and bases. Such compounds
are said to be amphoteric.
Strong acids have weak conjugate bases and strong
bases have weak conjugate acids. Water has the tendency to equalize
the strengths of all strong acids and strong bases, regardless of the strength
of the acid itself. This is known as the leveling effect. Acids
are limited to the strength of the H3O+ ions that they form
when they lose H+ ions when they dissolve in water. Likewise,
bases are limited to the strength of the OH- ions that they
form when they gain H+ ions when they dissolve in water.
There are numerous advantages to the Brønsted-Lowry
model of acids and bases:
-
Acids and bases can now be ions or neutral molecules.
-
Acids and bases can now be any molecule with at least one pair of nonbonding
electrons.
-
It explains the role of water in acid-base reactions; H2O accepts
H+ ions from acids to form H3O+ ions.
The H3O+ is present in all acid base reactions,
but is usually represented by simply H+. It is called
the hydronium ion.
-
It can be applied to solutions with solvents other than water and even
in reactions that occur in the gas or solid phases.
-
It relates acids and bases to each other with conjugate acid-base pairs
and can explain their relative strengths.
-
It can explain the relative strengths of pairs of acids or pairs of bases.
-
It can explain the leveling effect of water.
In 1923 Gilbert Newton Lewis took the definition of acids and bases further
and in a slightly different direction. He proposed:
-
Lewis acid - Any substance that can accept a pair of nonbonding electrons.
That is, it is an electron-pair acceptor.
-
Lewis base - Any substance that can donate a pair of nonbonding electrons.
That is, it is an electron-pair donor.
In the following example, the Al3+ ion acts
as an acid, accepting electron pairs from water which acts as a base, an
electron-pair donor. The two combine to form Al(H2O)63+,
an acid-base complex or a complex ion.

Although this definition may seem more difficult,
it is the least restrictive and can be used to explain the most challenging
acid and base reactions.
Reacting Acids and Bases
Strong acids and strong bases are those that ionize/dissociate
almost completely in a dilute aqueous solution. When a strong acid
of a given concentration in a dilute aqueous solution ionizes, the resulting
concentration of hydronium ions and the resulting concentration of the
conjugate base are equal to the original concentration of the acid.
Thus a 1M aqueous solution of hydroiodic acid exists as 1M H3O+(aq)
and 1 M I-(aq). Strong bases dissociate in
a similar manner. Since strong acids and bases ionize so completely,
any ionization from the water itself may be neglected in cases of ordinary
concentration of the acid or base. For concentrations less than
10-7 M, the ionization of water must be included.
Strength of an acid or base must not be confused with solubility.
It is possible for an acid or base to only be slightly soluble and yet still
be a strong acid or base. This would indicate that although, little
of the acid or base actually dissolves, most of the acid or base that does
dissolves is converted into ions. It is also possible for an acid
or base to be very soluble and yet still only be a weak acid or base indicating
that although most of the avid or base dissolves, very little is converted
into ions. Vinegar (acetic acid) is an example of an acid that is
very soluble yet it does not form a strong acid. Since acetic acid
is capable of dissolving so well, it can still be a very powerful acid
and care needs to be taken. A substance which forms ions readily
in water is called an electrolyte.
When acids contain more than one hydrogen atom (polyprotic
acids), they are capable of donating more than one hydronium ion. Thus
a strong polyprotic acid, such as sulfuric acid (H2SO4),
ionizes completely in water. However, the concentration of hydronium ions
will be twice the concentration of the original sulfuric acid. When the
pH of this solution is calculated, this higher concentration of hydronium
ions must be used.
Acid-base reactions are ubiquitous. In aqueous
solutions acids increase the hydrogen ion (H+) concentration.
On the other hand bases increase the hydroxide ion (OH-) concentration.
When an acid and a base react in an aqueous solution the H+
and OH- ions combine to form water. These ions thus "neutralize"
one another:
.
Really though we must include the hydronium ion:
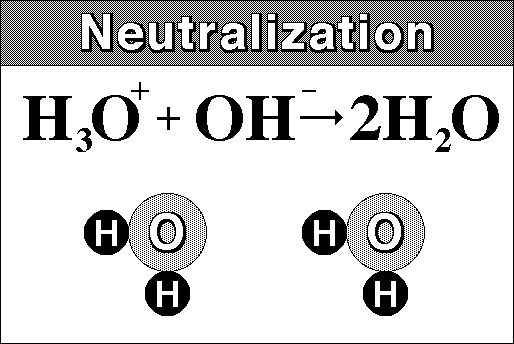
A salt is any compound which can be derived from
the neutralization of an acid and a base. A neutralization is a type
of double replacement reaction.
When acids and bases are mixed together, they can
either completely neutralize each other or partially neutralize each other.
In the case of complete neutralization, the moles of H+ and
OH- equal each other. A titration is a procedure
used in analytical chemistry to determine the amount or concentration of
a substance. In a titration one reagent, the titrant, is added to another
slowly. As it is added a chemical stoichiometric reaction occurs
until one of the reagents is exhausted, and some process or device signals
that this has
occurred. The purpose of a titration is generally to determine
the quantity or concentration of one of the reagents, that of the other
being known beforehand. In any titration there must be a rapid quantitative
reaction taking place as the titrant is added, and in acid-base titrations
this is a stoichiometric neutralization.
All acid-base titration reactions, as all acid-base
reactions, are simply exchanges of protons. The reaction could be
strong acid + strong base as in the case of HCl + NaOH above. Virtually
all acid-base titrations are carried out using a strong acid or strong
base. In most cases the strong acid or strong base is used as the
titrant. It is less common, but equally feasible, to place the strong
acid or strong base in the titration vessel and use the weak acid or weak
base as the titrant. A weak acid-weak base titration would have only
a small pH change at the equivalence point. This small change is
difficult to detect, and for this reason weak acid-weak base titrations
are uncommon.
One of the substances involved in a titration must
be used as a standard for which the amount of substance present is accurately
known. The standard can be present either in the form of a
pure substance or as a standard solution, which is a solution whose composition
is accurately known. A standard can be prepared in only two ways:
use a primary standard or standardize by titration
against some previously standardized solution. A primary standard
is some substance such as oxalic acid which can be precisely weighed out
in pure form, so that the number of moles present can be accurately determined
from the measured weight and the known molar mass. For example, we
might prepare a 0.1000 molar solution of primary standard oxalic acid by
weighing out exactly 0.1 moles of oxalic acid and diluting to one litre
in a volumetric flask.
The standard solutions used in an acid-base titration
need not always be primary standards. A standard solution which has
been prepared by quantitative dilution of a primary standard is an excellent
secondary standard solution. Secondary standards can also be prepared
by titration against a primary standard solution.
To titrate an unknown acid/base solution, take a
certain amount of the unknown solution and add a standard reagent of the
known concentration carefully until the neutralization reaction is completed.
This point where the number (or mole) of hydrogen ions and hydroxide ions
are equal is defined as the equivalence point. To determine
the equivalence point, scientists use an indicator or a pH meter.
First, you need to choose volume of an unknown acid
or base solution and put it in an Erlenmeyer flask. Second,
fill a buret with a standard reagent of known concentration and read the
initial volume of the solution. Of course, if you put an acid in
the Erlenmeyer flask, you need to put a base in the buret and vice versa.
A buret is a good apparatus for the determination of an equivalence point
in acid-base titration because you can accurately read the volume of solution
used. Third, add a couple of drops of an indicator in the flask for
titration. An indicator is a soluble dye that changes its color noticeably
over a fairly short range of pH. Different indicators show color
changes at different pH values and it is important to determine an indicator
to be used according to the expected equivalence point. Fourth, slightly
open the stopcock of the buret and add the standard reagent into the unknown
solution. Around the expected equivalence point of the titration,
you need to drop the solution very slowly and mix the solutions very well
because, around the equivalence point, just one drop of solution from the
buret can make a radical pH change in the mixed solution. If the
color of the solution in the Erlenmeyer flask changes, record the volume
of the solution in the buret and add a few drops of the solution
to make sure the equivalence point you found is correct. Finally,
using the data from your acid-base titration, you can calculate the concentration
of the unknown solution. The equation for this procedure is as follows.
(Here, M means molarity (concentration) of solution , and V means volume
of solution.)

For example, if you choose 10.0 mL hydrochloric acid
solution as a concentration-unknown solution and it takes 10.0 mL of 0.100
M sodium hydroxide solution to titrate, the concentration of the hydrochloric
acid is 0.100 M.
If you want to use a polyprotic acid or base (which
can donate two or more hydrogen or hydroxide ions per molecule) for titration,
you need to multiply the molarity of the solution by the number of hydrogen
or hydroxide ions it can donate per molecule. For example if H2SO4
were used instead of HCl, MAVA becomes 2MAVA.
End-point
A very typical observation that eventually becomes problematic deals with the misconception that neutralization always results in a neutral solution, but if this is true, how can an indicator with a transition point of anything but 7 indicate that equivalence has been achieved. The resolution to this issue relies on understanding that the salt formed from a neutralization is actually important. In the simplest sense, equivalence represents the point at which any acidic hydrogen ions have been reacted completely with any basic hydroxide ions forming water; however, the salt formed may further impact the water altering its pH. The salts formed can be neutral salts which have no impact on water since the concentration of H+ and
OH- present are not further altered, acidic salts which do impact water after equivalence such that the concentration of H+ present becomes greater than the concentration of OH- present after equivalence or finally, the salt may be a basic salt in which the concentration of H+ present becomes less than the concentration of OH- present after equivalence. This final pH due to the
salt is called end-point and typically not mathematically critical during a titration as long as the transition pH of the indicator used closely (as possible) matches the end-point and both are within the equivalence range of the titration. However, this does mean that even dissolving salt in water can alter pH when these salts split into ions or hydrolyze which is important when you realize just how many different salts are added to our water. This explains why farmers have to lime their land occasionally to restore soil pH due to the addition of fertilizer salts.
The key to this lies in the type of ions formed due to hydrolysis of the salt. If one of the ions is the conjugate ion of a weak acid or base, they will interact with water, resulting in a the formation of an basic or acidic solution respectively. For the most part, this can be simple to predict in prescribed lab conditions (why would you go out of your way to make it complicated) and this is needed to ensure selection of the proper indicator to identify equivalence during a titration. However, more complicated situations (aside from properly identifying the products of hydrolysis) do arise and cannot always be avoided.
Complicated Salt Hydrolysis
One challenge that arises can be due to the fact that both ions in the salt can be capable of interacting with water. This would mean the salt formed is mixing of a weak acid and a weak base to produce a salt made up of the two conjugate ions. Lets consider the salt, ammonium acetate, NH4CH3COO(s) which will hydrolyze in water quite readily since all ammonium salts are extremely soluble.
Since both these ions interact with water, predicting the outcome is not as straight forward. There is actually two ways to predict the outcome, both of which achieve the same answer. The simple method involves reacting ions in water by charge. Recall, water actually autolysis yielding hydrogen and hydroxide ions and since opposite charges attract, the process of combining all these ions is rather intuitive.
This would be possible since the reactions are weak equilibria and so are reversible and these reactions would actually represent the reverse of the typical acid/base equilibria for these reactions.
These two reversed reactions then result in a reduction of the amount of hydroxide and hydrogen ions in the water which could impact the overall pH. The task then becomes to determine whether the ammonium ion removes more or less hydroxide from the water compared to the amount of hydrogen ions removed by the acetate ions. This can be determined by comparing the Ka for the acetic acid which equals 1.79 x 10-5 with the Kb for the ammonium hydroxide (which is really aqueous ammonia) which equals 1.78 x 10-5. Since the Kb for the ammonium hydroxide (reverse reaction) is smaller (albeit slightly) than the
Ka for the acetic acid (reverse reaction), the ammonia ion removes slightly more
OH- from water compared to the removal of H+ by the acetate ions from the salt. This means the resulting salt solution will be slightly acidic. Essentially, more ammonium hydroxide reforms than acetic acid.
This all seems logical and can be used to determine the impact of dissolving such a salt in water, but in reality the odds of this happening in this manner is quite remote because the actual concentration of hydrogen and hydroxide in water due to the autolysis of water is extremely small. In reality the salt ions will actually be 10 million times more likely to interact with a water molecule in one liter of water than the appropriate ions. This direct water interaction is the more factually correct answer. In this case, the conjugate ions react with water according to a Brønsted-Lowry reaction and are not really the simple reverse of the original acid/base reactions.
These conjugate reactions require the conjugate K value which can be obtained by solving the
Kw =
Ka* Kb expression. So the
Ka for the first reaction = Kw / Kb = 1.0 x 10-14 /
1.78 x 10-5 = 5.618 x 10-10. Likewise, the Kb for the second reaction =
Kw / Ka = 1.0 x 10-14 /
1.79 x 10-5 = 5.587 x 10-10. Therefore, the real Ka> Kb which means more hydronium (or simply hydrogen ion) is released into the water, making the solution slightly acidic. So you can see, the same answer is obtained. This second method is more exact and the correct process, but the first method requires no math and so is often used for quick predictions.
An even more complex situation arises when one of the hydrolyzed ions from the salt is amphoteric (or even more complex, both ions are amphoteric). Consider the hydrolysis of sodium bicarbonate.
The positive sodium ion would combine with the hydroxide ion in the water but the sodium hydroxide would be a strong base and so it would not form and thus, cannot affect the pH of the water. However, the bicarbonate ion, HCO3-(aq), is amphiprotic in that it will both donate and accept a hydrogen ion so both cases need to be considered (both do in fact happen). In this case, the actual, direct interaction with water is the only method that can be used to determine the final answer.
The first reaction is a weak acid reaction with a Ka = 4.7 x 10-11 which is simply read from the Ka table. The second, weak base reaction is actually a conjugate reaction for the carbonic acid ion so the Kb =
Kw / Ka = 1.0 x 10-14 /
4.4 x 10-7 = 2.27 x 10-8. Since the Kb> Ka more hydroxide is released into the water than hydronium due to the simultaneous interactions of the bicarbonate ion with water molecules. Therefore, the water will end up being slightly basic. Exact pH values for both situations above would require ICE tables and quite a bit of math (much beyond our course).
Another interesting hydrolysis occurs with oxide salts. These salts can be metal oxides or non-metal oxides. Metal oxide salts tend to be ionic which means they dissociate in water to some degree. Consider calcium oxide.
The metal ion may interact with water if it is weakly bonded to with hydroxide ions present in the water; however, its impact will actually be small it can be ignored (unless exact pH calculations are needed). This is because the oxide ion formed has such an overwhelming impact on water. It is capable of chemically breaking down water by removing one hydrogen ion leaving behind a hydroxide ion while forming a new hydroxide ion.
The two reactions can be summed.
As a result, all metal oxides are basic salts. The more soluble the oxide, the more hydroxide produced. Furthermore, greater the degree of dissociation, the more hydroxide produced.
Non-metal oxides tend to be covalent and so the bond between the non-metal atom and the oxygen atom is quite strong. However, the oxygen still has a strong attraction to the hydrogen atoms in the water. As a result, non-metal oxides tend to undergo a synthesis reaction with water to form a weak acid. Consider carbon dioxide.
As soon as the weak acid forms, it further reacts with water to release hydronium ions.
The two reactions can be summed.
As a result, all non-metal oxides are acidic salts. The more soluble the oxide, the more hydronium produced.